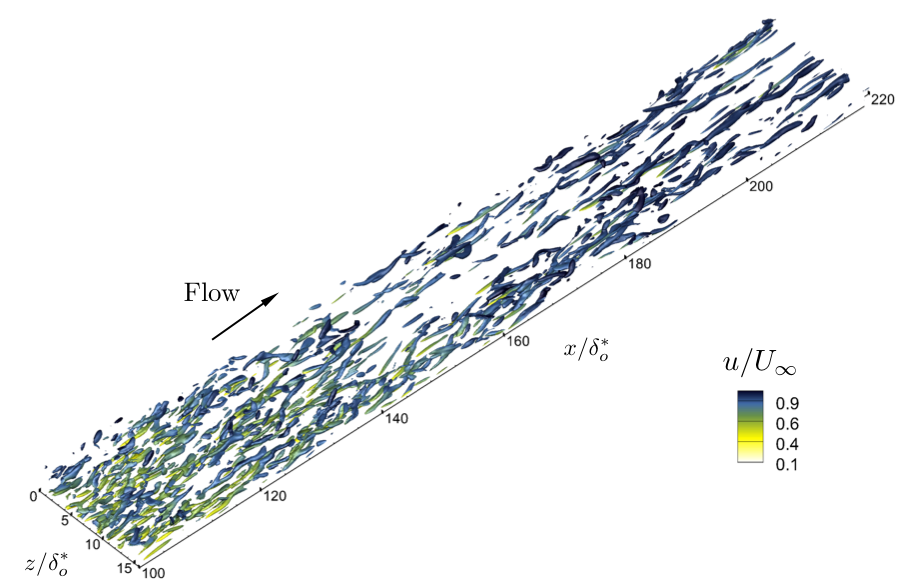
Boundary layer with pressure gradients
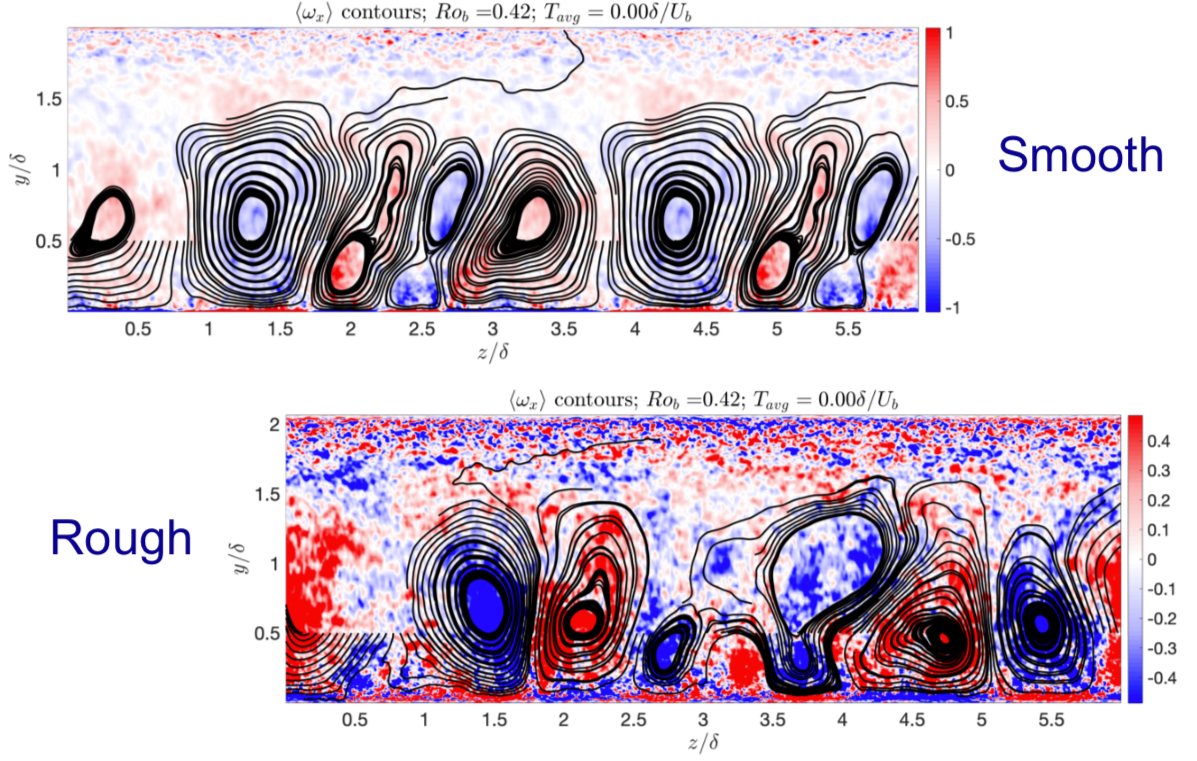
Rotating channel flows
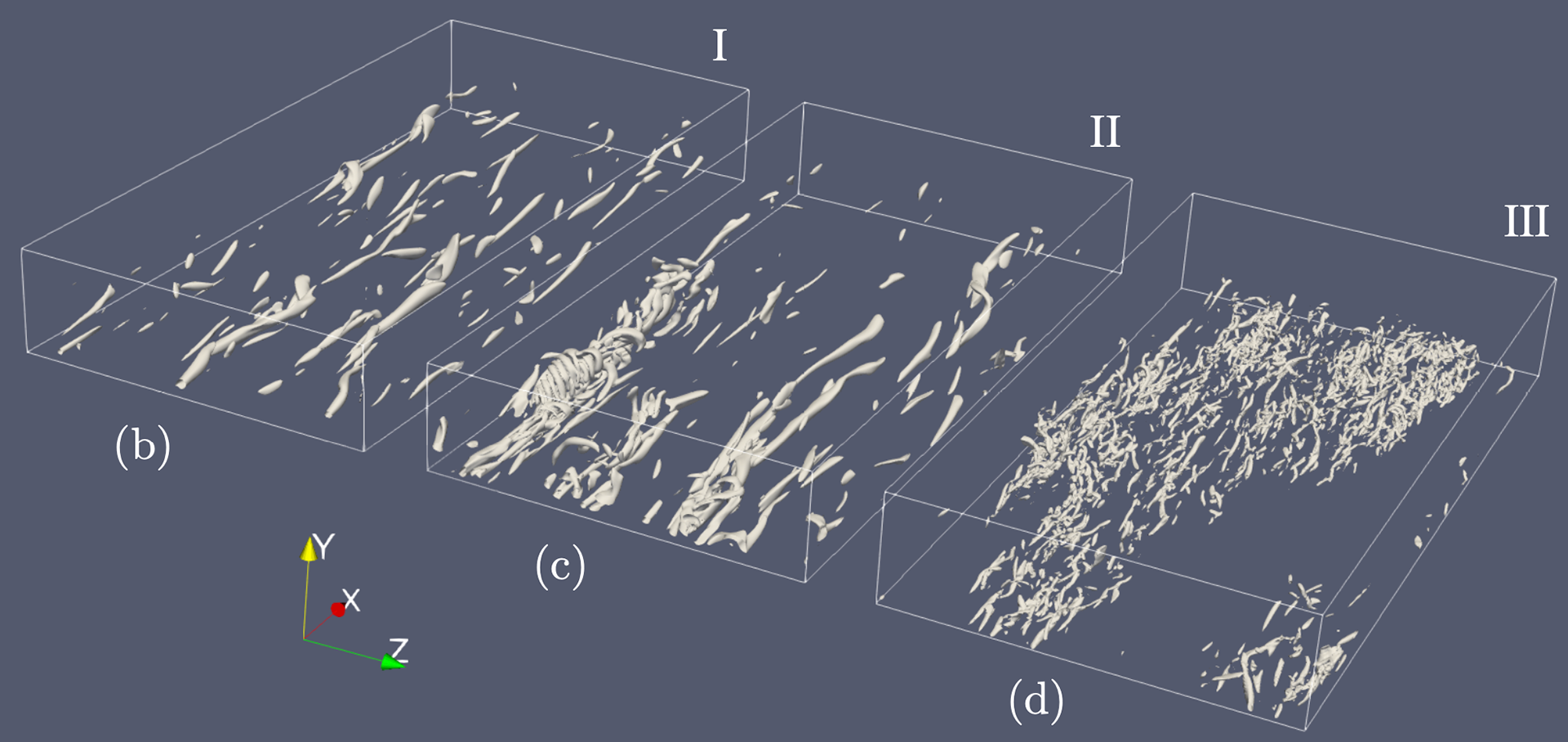
Stages of an accelerated turbulent channel
Non-equilibrium wall-bounded turbulence
Turbulent flows are ubiquitous, and consist of a wide range of length and velocity scales; resolving the smallest scale in a DNS is difficult. In practical design and analysis, the constraints of time and computational recourses require the use of turbulence models to approximate the effects of the small scales. However, model errors are inevitable, since the models are calibrated in simpler scenarios (e.g., homogenous, equilibrium state with simple wall geometries) than those in reality. DNS of a realistic flow is a useful tool to quantify this error, and aid model improvement.
Turbulent boundary layers in reality are characterized with local strong pressure gradients; examples include flows around airfoils or atmospheric boundary layers above complex terrains. In cases of strong favorable pressure gradients, for instance, the boundary layer undergoes a “relaminarization” process, opposite to the model prediction that an increase of mean-flow kinetic energy leads to higher turbulent kinetic energy (an assumption used in common engineering turbulence models). High-resolution simulations help identify the underlying physics, and where the models go wrong.
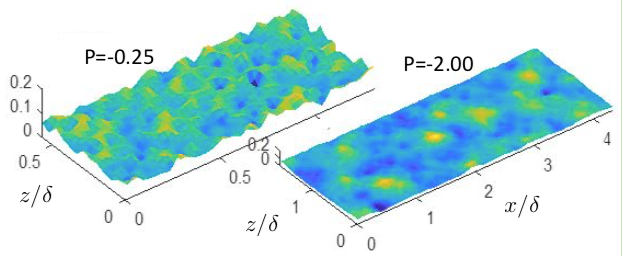
Synthesized fractal roughness
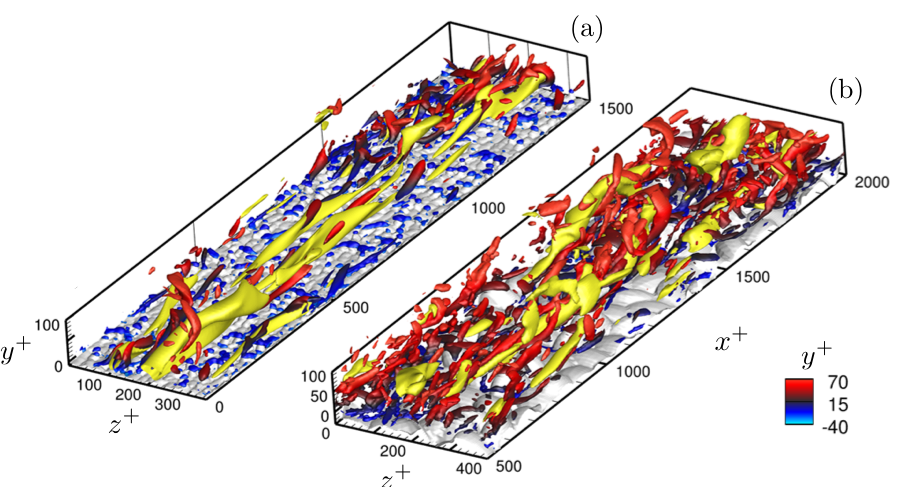
Sink flow with surface roughness
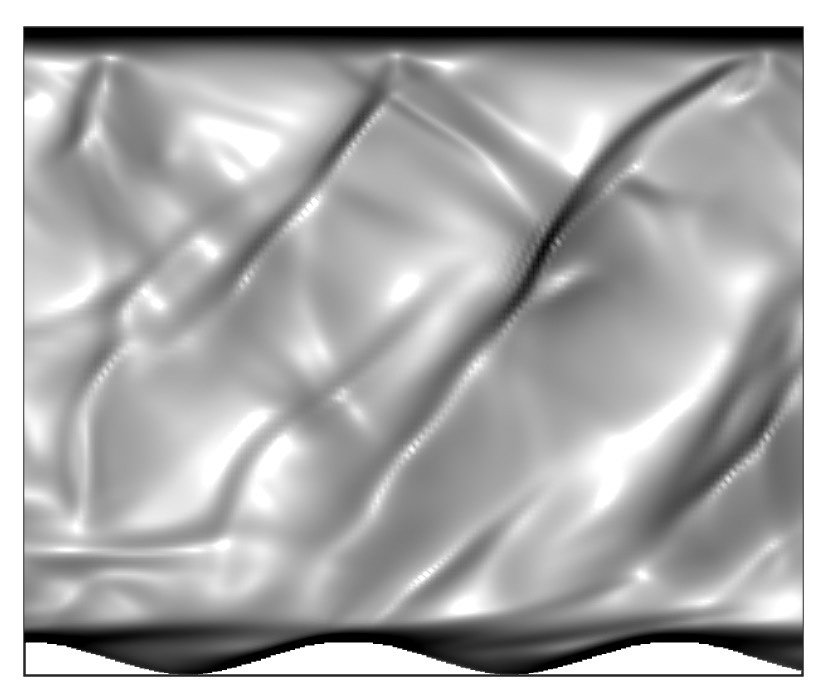
Compressible turbulence on rough wall
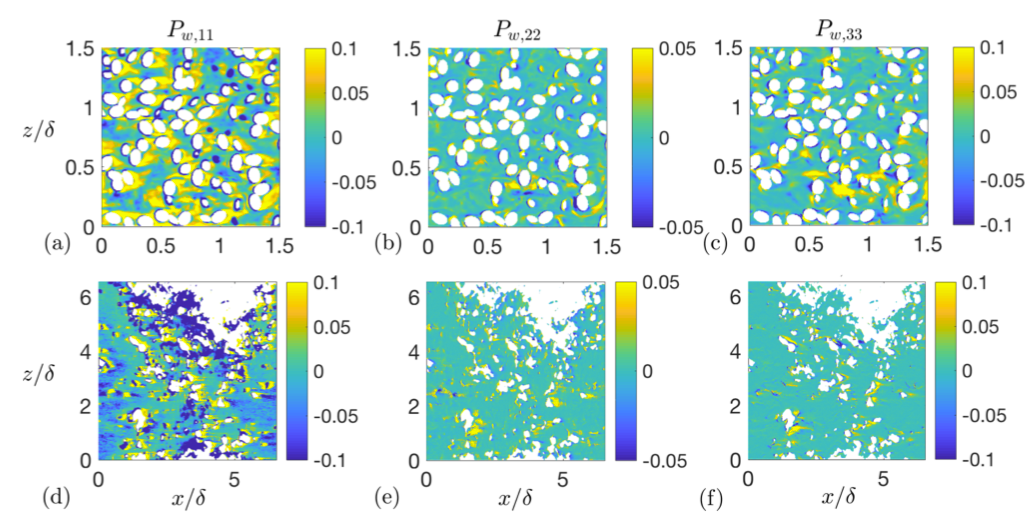
Roughness-induced TKE production
Roughness effects on turbulence
Roughness is another widespread phenomenon in real-world boundary layers, such as airplane wing icing, turbine blade erosion, and in a larger scale, plant or ocean canopies. We aim to improve fundamental understanding of non-equilibrium turbulent flows close to rough surfaces and to develop single-point closures. We established an extensive roughness-resolved DNS database that contains a wide range of turbulent flows over many different roughness geometries. These data were used to identify the role of roughness and its topography in modifying turbulent statistics and structure. In particular, results revealed similarity of the roughness sublayer velocity in non-equilibrium flows, which is of important implication in developing closures for non-equilibrium turbulence. Leveraging the database, my group developed machine-learning (ML) techniques to determine roughness-specific model coefficient values for Reynolds-averaged Navier-Stokes (RANS) closures for almost arbitrary roughness. We developed new representations of roughness in turbulence models and tested them in equilibrium and selected non-equilibrium flows. Compared to existing roughness treatments in RANS closures, the new models offer advantages such as preserving near-wall stress balance and the potential of describing separated boundary layers. My group has also developed and validated numerical methodologies to simulate complex roughness in compressible turbulent flows and used it for preliminary studies of roughness geometrical effects.
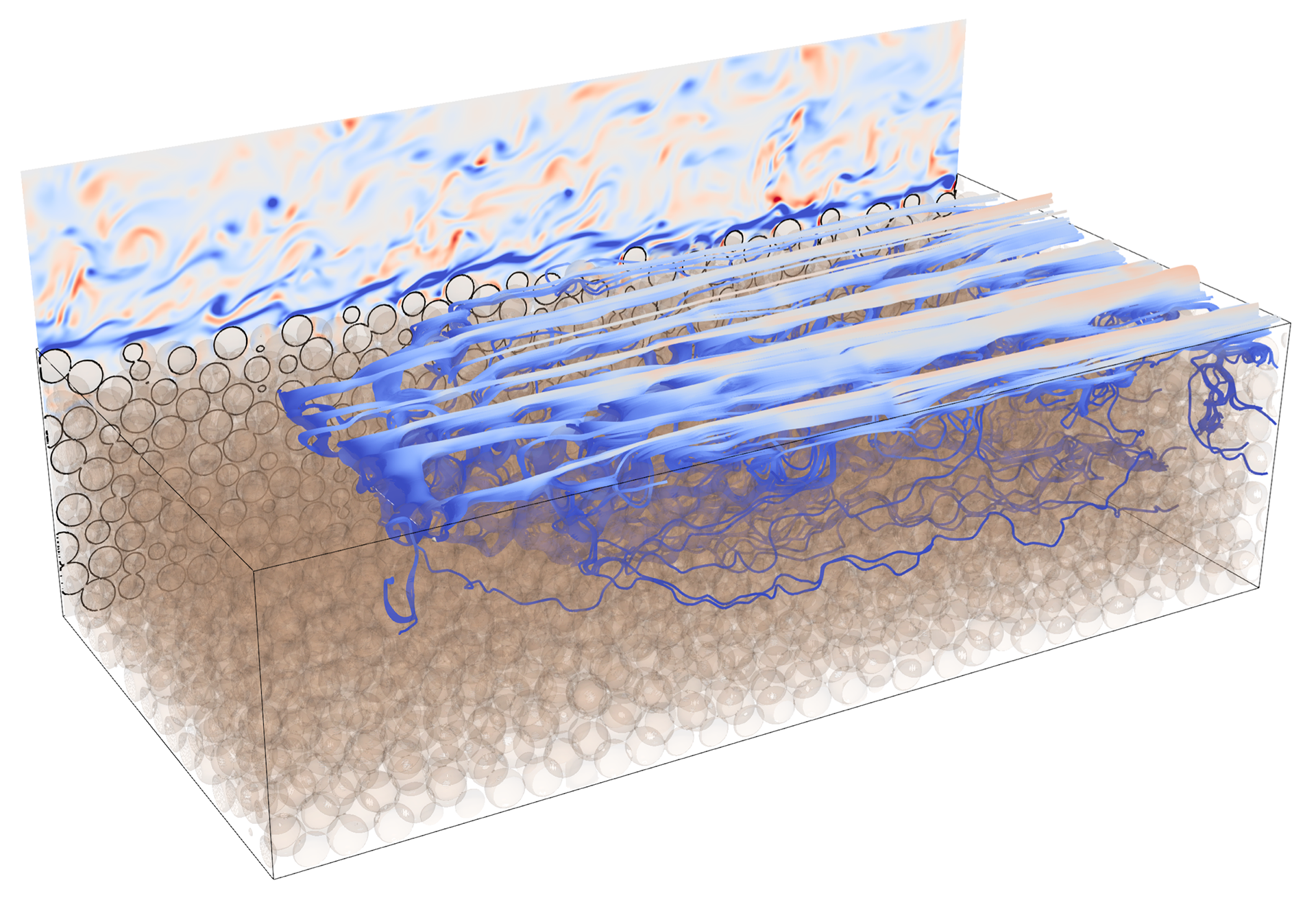
Pore-resolved simulation
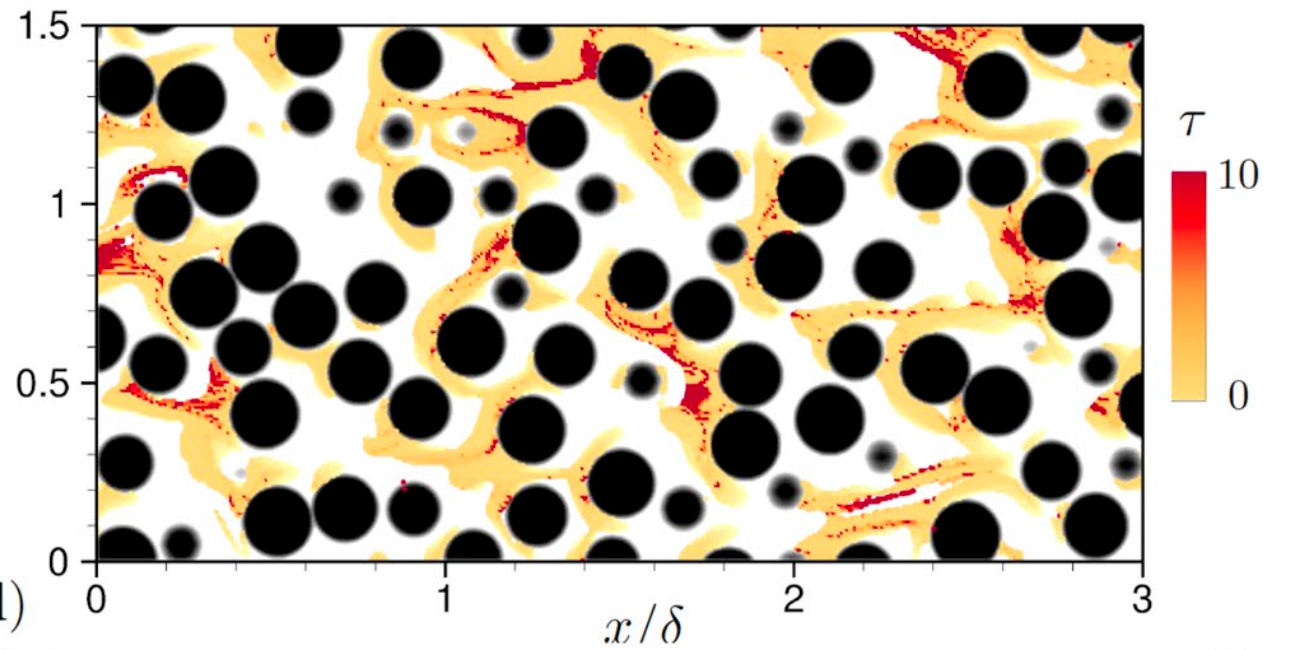
Grain roughness determines transit time.

Exchange across a rough bedform
Permeable wall turbulence and hyporheic exchange
We characterize hyporheic exchange (i.e. the exchange of water and solute between a stream and the sediment beneath it) based on sediment-pore-resolved numerical simulations of the turbulent stream-sediment system. For transport predictions at the scale of river reaches, existing analytical or computational models are known to yield order-of-magnitude accuracy at best, notably attributed to a major knowledge gap of processes at the scale of individual pores. We conducted DNS simulations of mass and momentum transports across the sediment-water interface (SWI) of a porous bed with different topographies, including large-scale bedforms and grain-scale roughnesses. Small-scale exchange processes are characterized at scales far below the resolutions in most existing field/lab measurements and computation models. We discovered that grain roughness not only modifies the bed friction but also induces additional roughness-scale interfacial pressure variation (i.e. roughness pumping) similar to the known pumping effects of bedforms. On a macroscopically "flat" bed, we showed that roughness surprisingly incudes significant large-scale subsurface flow paths and long transit times of water inside the bed, which are ignored in existing models of hyporheic exchange. However, when superimposed on a bedform, grain roughness appears to act differently to modulates the exchange induced by the bedform. The long term goal is to characterize transport of both conservative and reactive solutes across streambeds with multiscale topographies.
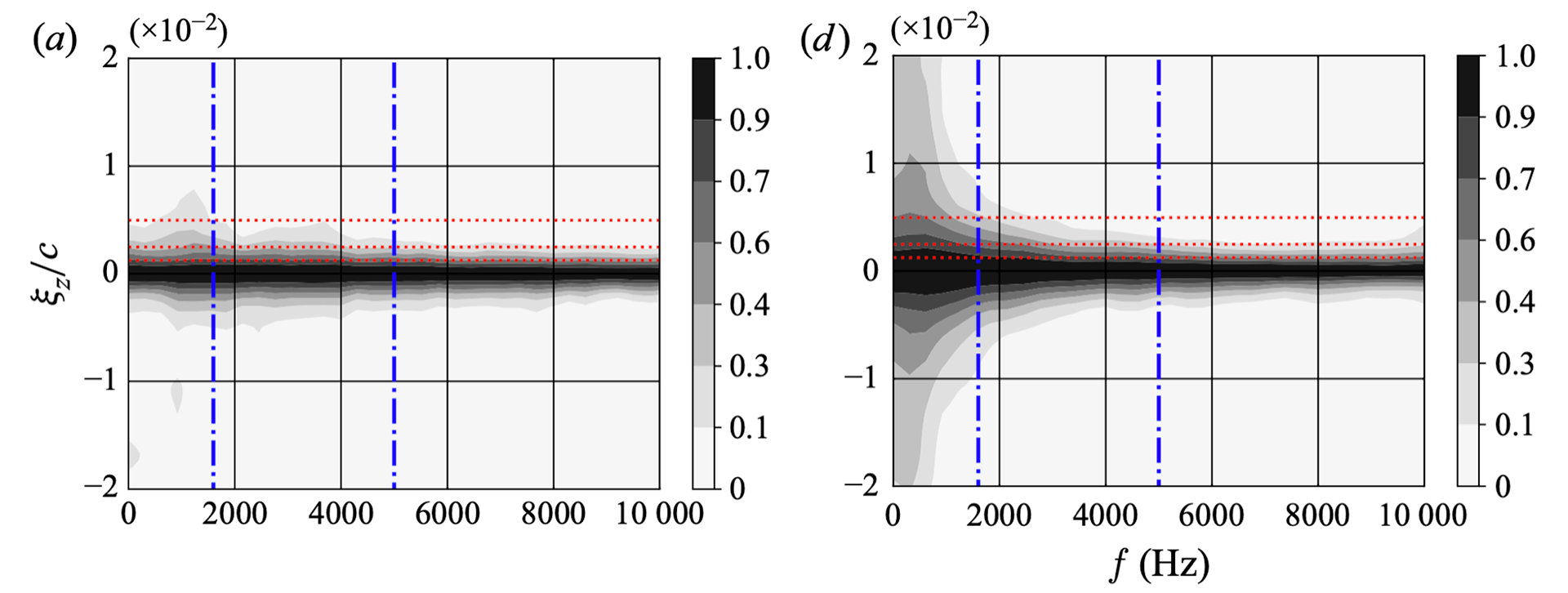
Wall pressure characteristics under zero (left) and adverse (right) pressure gradients.
Turbulence-induced noise and fan application
We aim to develop fast-prediction tools for fan acoustic and efficiency characteristics, informed by simulations of idealized fluid flows within fans. Our group is part of the Consortium III for the Development of Ultra-High Efficiency Quiet Fans, formed by 4 universities and 4 industrial partners. The topic is on understanding and modeling of noise generation in non-equilibrium turbulent boundary layers typical on a fan blade. We demonstrated quantitatively that efficient DNS simulation techniques for wall-bounded flows (i.e. minimal-span simulations and idealization of curved-wall flow with a flat-plate one with matching pressure gradient) can be used to approximate real flows around fan aerofoils. We collected an extensive database of DNS, LES and measurements and develop a generalized wall-pressure-spectrum closure (an essential part of aero-acoustics prediction) for both equilibrium and non-equilibrium boundary layers.